Research Interests
Hookworms – a neglected tropical disease
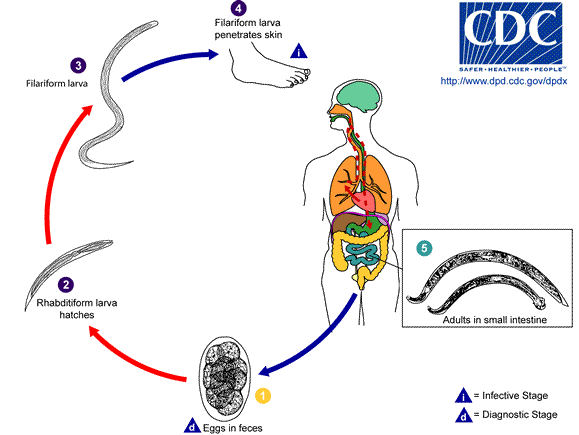
Hookworms are one of the soil transmitted helminths (STH). People become infected when they come in contact with the infective stage in the soil. After penetrating the skin, the larva migrates via the lung to the small intestine, where it matures and attaches to the small intestine to feed on blood. Heavy hookworm infections cause anemia, which is especially devastating in children and pregnant females. The World Bank estimates that more than 20% of the disability-adjusted life years (DALYs) lost from communicable diseases among school-age children living in endemic areas are a direct result of hookworms and other intestinal nematodes. Current control strategies are limited to periodic de-worming of infected individuals, which is limited by rapid re-infection rates and the development of drug-resistant worm populations. The development of new drug targets and vaccine antigens will require a better understanding of hookworm biology, particularly the infective process.
Projects
Anthelmintic resistance in hookworms
Currently, hookworms and other parasitic nematodes are controlled by treatment with anthelmintic drugs that kill the mature worms in the host. Efforts are underway to control morbidity by mass drug administration (MDA) using the anthelmintics albendazole (ABZ) and mebendazole (MBZ), members of the benzimidazole (BZ) class of drugs, ivermectin (IVM), a macrocyclic lactone derivative used for lymphatic filariasis control, and pyrantel (PYR), commonly used for hookworm in children. While relatively effective, people in endemic regions are rapidly re-infected, necessitating treatment on an annual or biannual schedule. Heavy exposure to BZ anthelmintics has selected populations of veterinary PNs (including hookworms) that are no longer susceptible to the drugs, rendering this class of anthelmintics useless in many areas of the world. The rapidity with which anthelmintic resistance (AR) developed to these drugs suggests that increasing the selective pressure on human helminths will rapidly generate resistant worm populations as well. As MDA programs scale up, detecting emerging AR will become critical to avoid losing the most effective treatments.
Understanding the mechanism of AR in hookworms requires access to confirmed resistant worm populations. Multidrug resistance has emerged in populations of the canine hookworm Ancylostoma caninum, originating in rescued greyhounds, but now occurring in multiple breeds throughout the US. Previously, we isolated the first known isolate of the canine hookworm Ancylostoma caninum resistant to BZ and IVM. Recently we identified a second isolate resistant to BZ, IVM and PYR. We are using genomics and in vitro assays to probe the mechanism of resistance to these drugs. Identification of the genetic mutations underlying resistance will allow us to develop tools to monitor the emergence of resistance in natural hookworm populations undergoing MDA in time to prevent its spread.
Anthelmintics from natural products
People in endemic regions must be treated annually or biannually due to rapid re-infection following treatment. As MDA programs proliferate, resistance is predicted to arise in human hookworms as it has in livestock parasitic nematodes. Therefore, the need to develop new drugs is critical to ensure continued control of hookworm. Momordica charantia (MC), or bitter melon, is globally cultivated for food and its numerous health benefits. MC has anthelmintic activity against Caenorhabditis elegans and other free-living nematodes, and ethnomedical practitioners in Haiti and Jamaica use MC tea to treat intestinal worms. Our lab has shown that MC aqueous extract (MCE) of plant leaves causes permanent inhibition of feeding in Ancylostoma caninum infective third-stage larvae (L3). Our preliminary data indicate that ingestion of MCE by parasitic fourth-stage larvae (L4) and adult hookworms is lethal, and that a short exposure stops esophageal pumping prior to death. Identification of the anthelmintic compound(s) in MCE could provide a safe and effective de-worming treatment. Moreover, the widespread use of MC as a foodstuff and medicinal plant suggests a favorable safety profile that could facilitate rapid deployment of a new anthelmintic. Development of MCE as an anthelmintic begins with identification of the active component(s). We are working with collaborators at GW and Virginia Tech to isolate the active component(s) of MCE by using standard natural products chemistry techniques, elucidate the chemical structure of the active compound(s) with analytical spectrometry tools, determine selectivity and toxicity, and test the effect on hookworm infections in vivo.
Molecular mechanism of hookworm host specificity
Identifying the molecular determinants of host specificity represents a long-standing goal in parasitology. Why are some parasite species capable of infecting and reproducing in multiple host species, whereas closely related parasites can only colonize a single species? What is the molecular mechanism of host specificity? Hookworms offer an excellent model for answering these questions. Congeneric Ancylostoma hookworms show a range of host specificities, from strict specialists to generalists. The hookworm A. caninum is a strict specialist, infecting only canids, whereas the generalist A. ceylanicum naturally infects dogs, cats, and humans, and infects and reproduces in golden hamsters in the lab. The human hookworm A. duodenale, which can infect dogs under certain conditions, is an example of a species with intermediate host specificity. Evidence suggests that host specificity is mediated immunologically. We are investigating the molecular determinants of host specificity in hookworms using genomic and immunological approaches.
Other interests
Hookworm infective process
During infection of the definitive host, the third-stage infective larva (L3) of hookworms encounters a host-mediated signal that re-activates its arrested developmental programs. Execution of this developmental program culminates in the establishment of a successful parasitic relationship. This transition from the free-living L3 to the parasitic L3 is therefore a critically important, but poorly understood, event in parasitism. We have developed an in vitro system, in which larval feeding is used as a marker of activation, to model the early events of this “transition to parasitism” in hookworms. This process is analogous to recovery from the developmentally arrested Caenorhabditis elegans dauer stage. An insulin/insulin growth factor signaling (IIS) pathway mediates dauer recovery by negatively regulating the forkhead transcription factor DAF-16, thereby causing the expression of the normal developmental program. Our data indicate that hookworms share several IIS pathway steps with C. elegans, and that inhibition of IIS prevents hookworm L3 activation. We have also shown that the DAF-16 molecule from the hookworm Ancylostoma caninum (Aca-DAF-16) binds to the same regulatory sequence as does the Cel-DAF-16, and can rescue C. elegans daf-16 mutants when expressed as a transgene.
Entomopathogenic nematodes as models of the hookworm infective process
Despite important progress in the field of innate immunity, our understanding of host immune responses to parasitic nematode infections lags behind that of responses to microbes. Similarly, little is known about the molecules nematodes use during infection. The major factor limiting progress in these areas is the obligate requirement for a vertebrate host. The developmentally arrested dauer stage of the free-living nematode Caenorhabditis elegans is analogous to the infective stage of many important parasites, and recovery from dauer has been used as a model for resumption of development during infection. The availability of powerful molecular and genetic tools makes it a useful surrogate for studying some aspects of parasitic nematode infection. However, C. elegans is not a parasite, and the dauer stage is facultative and not obligatory as in PNs. Therefore, a parasitic equivalent of C. elegans would allow more relevant investigations into parasitic nematode infection mechanisms. The insect parasitic nematodes Heterorhabditis bacteriophora and Steinernema hermaphroditum offer great potential as models. With their endosymbiotic bacteria, these nematodes invade multiple insect species, including Drosophila melanogaster fruit flies, which it kills and exploits as a food source for the development of several nematode generations. When the insect cadaver is exhausted, infective (dauer) juveniles (IJs) exit and seek a new host to infect. The obligatory dauer infective stage renders these nematodes as a more representative model of parasitic nematode infection than C. elegans, and the ability to culture the life cycle on plates will allow studies of the molecular events occurring during infection of the host that are inaccessible using vertebrate hosts. The Drosophila-nematode systems are considered ideal models to investigate simultaneously the molecular basis of parasitic nematode pathogenicity and host immune function.